The make-up
of the brain
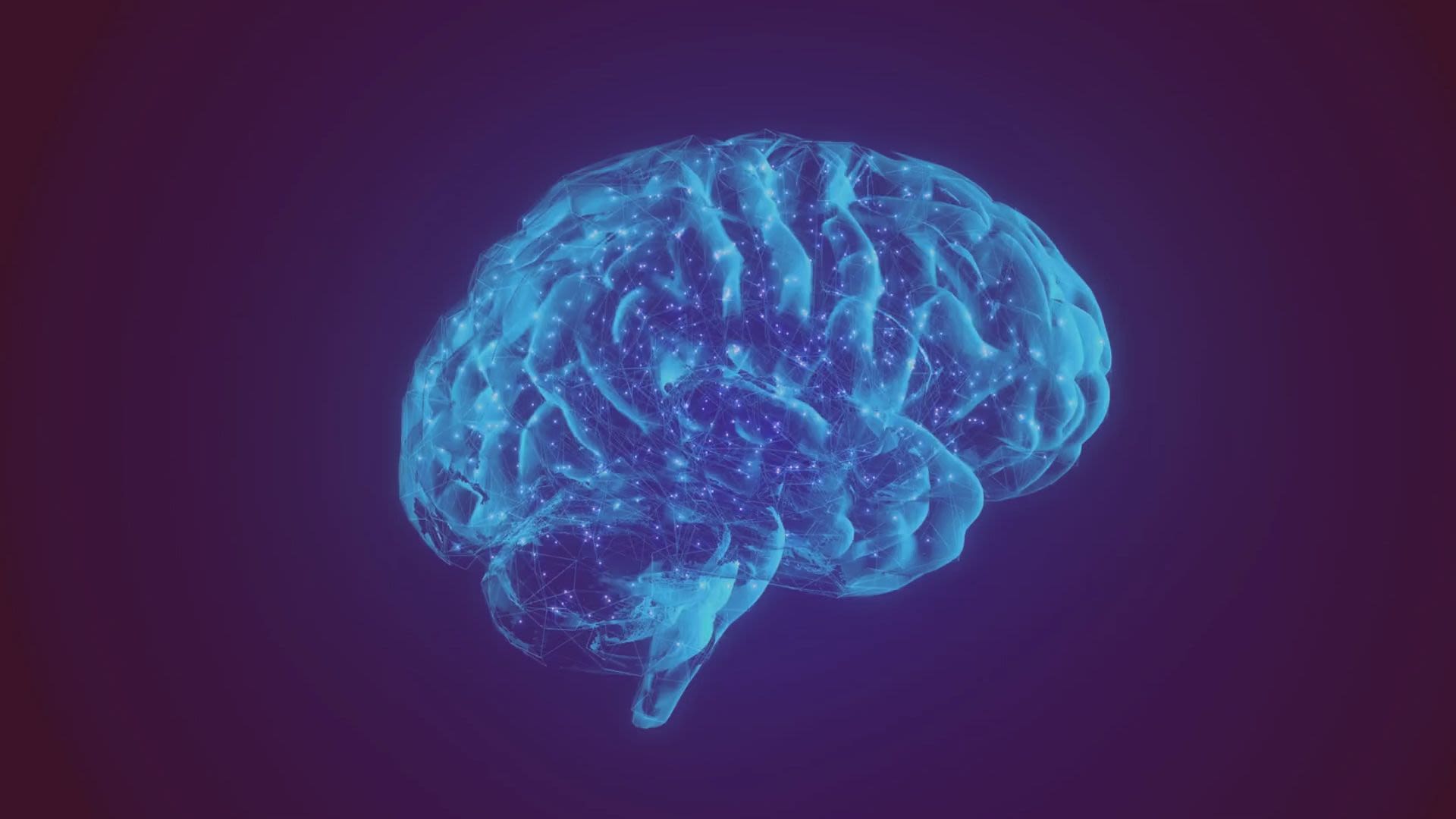
As the control system of the body, the brain regulates the functions and behaviour that shape how we experience the world, it influences our personality and moods, and determines different aspects of mental wellbeing.
From building understanding of cellular-level brain structure fundamentals, to investigating the big questions surrounding consciousness and brain enhancements, Oxford’s researchers are continuing to uncover the secrets of this complex organ.
Anatomy of the brain
The human brain is the command centre that controls the body’s responses to sensory inputs from the environment.
Identifying functions of different structures of the brain allows researchers to better understand underlying causes of neuropsychiatric conditions and develop targeted treatments.
The cerebral cortex is a thin layer made up of grey matter, where neurons are densely concentrated, covering the brain and controlling high-level cognitive functions.
The cerebral cortex makes up about 80% of the brain’s volume and is responsible for many complex phenomena, including perception, thought, language, attention and memory.
Researchers from across the University of Oxford, including the Department of Physiology, Anatomy and Genetics’ Molnár and Vyazovskiy groups, are investigating one of the most interesting questions in modern neuroscience, the role of the cerebral cortex in the processes behind sleep.
‘The cortex is a highly complex structure, both anatomically and functionally, and is therefore difficult to study; and this is why we think its role in sleep control was previously overlooked. The effects on sleep of cortical silencing offers a novel and fresh perspective on the mechanisms of sleep control, and has the strong potential to transform the field of sleep neurobiology.’
The prefrontal cortex is the part of the cerebral cortex at the front of the brain.
A new study from researchers at Oxford’s Experimental Psychology Department has highlighted the crucial role of the prefrontal cortex in future planning.
The researchers tracked a brain activity pattern in the anterior lateral prefrontal cortex to find that people have an advance sense of how well they will do at a task even before they try it.
A team of neuroscientists at Oxford, funded by The Wellcome Trust, investigated the role of the prefrontal cortex in apathy, suggesting the structure of this brain region impact levels of motivation.
‘We know that in some cases people can become pathologically apathetic, for example after a stroke or with Alzheimer's disease. Many such patients can be physically capable. Yet they can become so demotivated they won’t be bothered to care for themselves, even though they're not depressed. By studying healthy people, we wanted to find out whether any differences in their brains might shed light on apathy.’
A new study from the Department of Physiology, Anatomy and Genetics’ Bruno Group is challenging our perceptions of how the different regions of the cerebral cortex function.
Research team Dr Rebecca Rabinovich, Dr Daniel Kato and Professor Randy Bruno focused on investigating the ‘quiet’ cells of the somatosensory cortex, primarily associated with touch. The results revealed new evidence for how our brains handle surprise, indicating an important and previously unidentified role across all the major cortices.
The cerebrum is the foremost part of the brain and is involved in the functions associated with intellectual activities.
The cerebrum is split into two almost identical hemispheres, each controlling different functions.
A new study, led by researchers at the University of Oxford and funded by the Medical Research Council and Wellcome, has for the first time identified regions of the genome associated with left-handedness in the general population and linked their effects with brain architecture.
Using detailed brain imaging, researchers Dr Akira Wiberg, Professor Gwenaëlle Douaud and Professor Dominic Furniss found that in left-handed participants, the language areas of the left and right sides of the brain communicate with each other in a more coordinated way.
‘Around 90% of people are right-handed, and this has been the case for at least 10,000 years. Many researchers have studied the biological basis of handedness, but using large datasets from UK Biobank has allowed us to shed considerably more light on the processes leading to left-handedness.’
The cerebellum coordinates voluntary muscle movement such as posture and balance.
The brain stem relays information to and from the body and controls vital, involuntary functions such as breathing and swallowing.
The spinal cord follows instruction from the brain to produce movement in the body.
The frontal lobes of the brain perform executive functions such as decision making and problem solving.
Researchers from Oxford’s Experimental Psychology Department used MRI imaging to identify a brain area unique to humans. The ventrolateral frontal cortex is involved in some of the most advanced planning and decision-making processes, which may help explain our unique cognitive abilities. They were funded by the UK Medical Research Council
‘We tend to think that being able to plan into the future, be flexible in our approach and learn from others are things that are particularly impressive about humans. We've identified an area of the brain that appears to be uniquely human and is likely to have something to do with these cognitive powers.’
The parietal lobes process and integrate sensory information from the outside world, and are involved with functions such as limb movement and spatial awareness.
The occipital lobes are involved in visual processing.
The temporal lobes are involved in audio processing.
Findings from a computational modelling study from the Department of Physiology, Anatomy and Genetics’ King Group demonstrates that the way sounds are transformed from the ear to the brain’s auditory cortex may be simpler than expected.
The team, led by Dr Nicol Harper, examined how well different models of the ear and auditory nerve could be used to predict responses of brain cells in the primary auditory cortex.
‘Understanding the computations performed by the auditory pathway, as well as providing insight into brain function in general, will aid us in developing better hearing aids and ear and brain implants to help people with hearing loss.’
Researchers at the Oxford Centre for Functional Magnetic Resonance Imaging of the Brain identified the brain area directly related to the intensity of pain, known as the dorsal posterior insula.
‘We have identified the brain area likely to be responsible for the core, 'it hurts', experience of pain.’
Using a new brain imaging technique to perform an imaging study of 17 people, the team, led by Professor Irene Tracey, were able to show that activity in only one brain area, the dorsal posterior insula, reflected the participants' experience of pain.
Led by Adam Packer, Principal Investigator of the Packer group, a collaboration of Oxford researchers has shown that a little understood region of the brain called the claustrum may play an important role in how we experience pain.
Researchers from the Department of Physiology, Anatomy & Genetics, the Nuffield Department of Clinical Neurosciences and Experimental Psychology reviewed studies of patients with lesions in the claustrum, which although rare, show cognitive impairments and seizures. They also uncovered an under-appreciated link between the claustrum and pain.
This little understood area of the brain may be the next frontier in improving outcomes for brain damage patients.
The limbic system is a collection of structures involved in memory, emotions and motivation. In particular, the amygdala plays a role in decision making and emotional responses (such as fear, anxiety and aggression).
The amygdala is primarily associated with emotional processes and the “fight, flight or freeze” responses.
Associate Professor Miriam Klein-Flügge and colleagues looked at brain connectivity and mental health data from nearly 500 people to examine the connectivity of the amygdala. They also used questionnaires to capture information on well-being from a large group of healthy people.
The study shows how the improved level of detail about both brain connectivity and wellbeing made it possible to characterise the exact brain networks that relate to these distinct aspects of mental health.
‘Understanding how changes in the brain relate to changes in well-being is an important step in the journey towards more targeted mental health treatments.’
The hippocampus is involved in the processing and storage of short-term memory.
The hypothalamus plays an important role in hormone production and controls body processes such as temperature regulation, blood pressure and fluid balance.
The thalamus relays information between the cerebral cortex and other parts of the brain.
A 2016 study from Oxford’s Experimental Psychiatry Department was among the first to highlight the role of the thalamus in decision-making.
Research from the Department of Physiology, Anatomy and Genetics Molnár Group, led by Professor Zoltán Molnár, has given new insights into how the brain rewires after sensory loss, and the role the thalamus plays in this process.
‘The brain compensates for sensory loss on many different levels, especially early sensory loss. During development, the sensory signals shape our brain and determine how large an area we dedicate to certain functions. Our study shows a new mechanism that was not considered before.’
The pituitary gland controls glands throughout the body and regulates hormones.
Basal ganglia are a group of nerve clusters involved with voluntary movement. Parkinson’s disease affects this area.
The researchers, including Professor Peter Brown of the Medical Research Council Brain Network Dynamics Unit, demonstrated that the way activities in the basal ganglia combine to produce a physical effect could accurately be described mathematically, making make it possible to produce devices that regulate the force or speed of the movements.
‘Our results suggest how the basal ganglia help to direct parts of the brain controlling muscle responses, and how this might go wrong in Parkinson’s disease. The accuracy with which force could be predicted raises the possibility of producing high-performance control signals for brain-controlled devices, offering the fine-tuning that would be necessary for more delicate and complex tasks like picking up objects.’
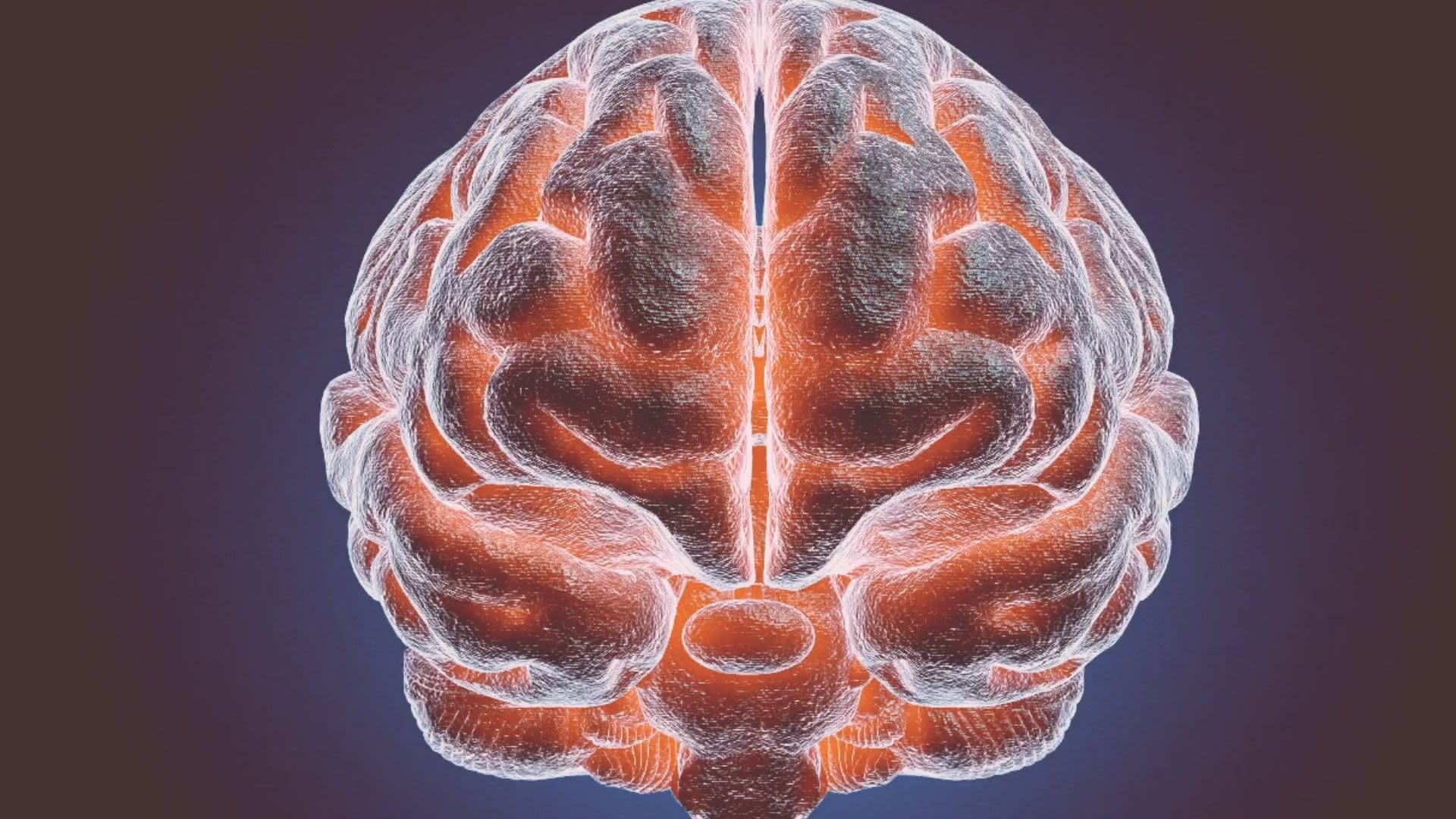
Neurons & Synapses
The brain is packed full of billions of nerve cells, or neurons, which form interconnected pathways, joined via synapses (or gaps) between adjacent neurons. Electrical messages travel along the axon of one neuron and pass to the next neuron across the synapse, with the aid of chemical messengers, or neurotransmitters, these interconnecting neural pathways enable both simple and complex responses to be coordinated such as movement, hormone release, behaviour and learning.
Understanding the specific characteristics of neuron responses is a major goal in neuroscience.
Researchers at the University of Oxford, working with colleagues from Princeton University, have developed a new way to test the theory that active neurons can change what they signal in the world, rather than keeping a stable correspondence to things. This is providing new insights into how the brain encodes information about the world.
Using a simple rule called 'Hebbian plasticity' to simulate flexibly coding neurons, Associate Professor Sanjay Manohar and his team were able to produce a distinctive signature indicating when the neuron will become active.
Proper development of neurons is key to how our brains encode and store information about our surroundings and experiences, and can inform an individual’s future mental health. The role of proteins in early stages of brain development has until recently remained unknown.
Using high-resolution X-ray crystallography, the team of Professor Elena Seiradake from Oxford’s Department of Biochemistry was able to find out more about how the proteins on the surface of nerve cells hold together. Working together with the teams of Matthieu Chavent at the University in Toulouse, and Rüdiger Klein at the Max-Planck Institute of Neurobiology in Germany, the researchers identified how these proteins guide migrating nerve cells as the brain forms, enabling them to further investigate their impact on brain development.
The Department of Physiology, Anatomy and Genetics’ Lak Group, with funding from a new ERC Starting Grant, will investigate neural circuits to address a fundamental question in neuroscience: How does the brain learn to make efficient decisions in an uncertain world?
With over £1.25 million of funding Dr Armin Lak and his team of researchers will embark on a new project investigating neural bases of long-term learning under uncertainty.
‘This is an uncharted space of research entailing significant risk and promising scientific breakthrough.’
Due to their role underpinning learning and memory, neuronal and synaptic activity are a major focus of neuroscience research.
In Oxford’s Department of Pharmacology, the group led by Dr Tim Viney and Professor Peter Somogyi, has discovered a new type of neuronal pathway that may be important in memory. The group looked at the precisely timed sequences nerve cells are activated in for recalling episodic memories, and identified the degradation of these rhythms, such as through neurodegeneration, to cause memory loss.
When neurons in the brain communicate with each other, they generate synchronised electrical activities known as brain waves. But what is the function of these brain waves? Can we see them? What happens if these collective activities go out of sync?
The Medical Research Council Brain Network Dynamics Unit and Oxford Sparks teamed up to produce The Symphony of the Brain, where Demi Brizee, a DPhil student, introduces us to the fascinating world of brain waves, and explains how a better understanding of them could lead to new therapies for neurological conditions.
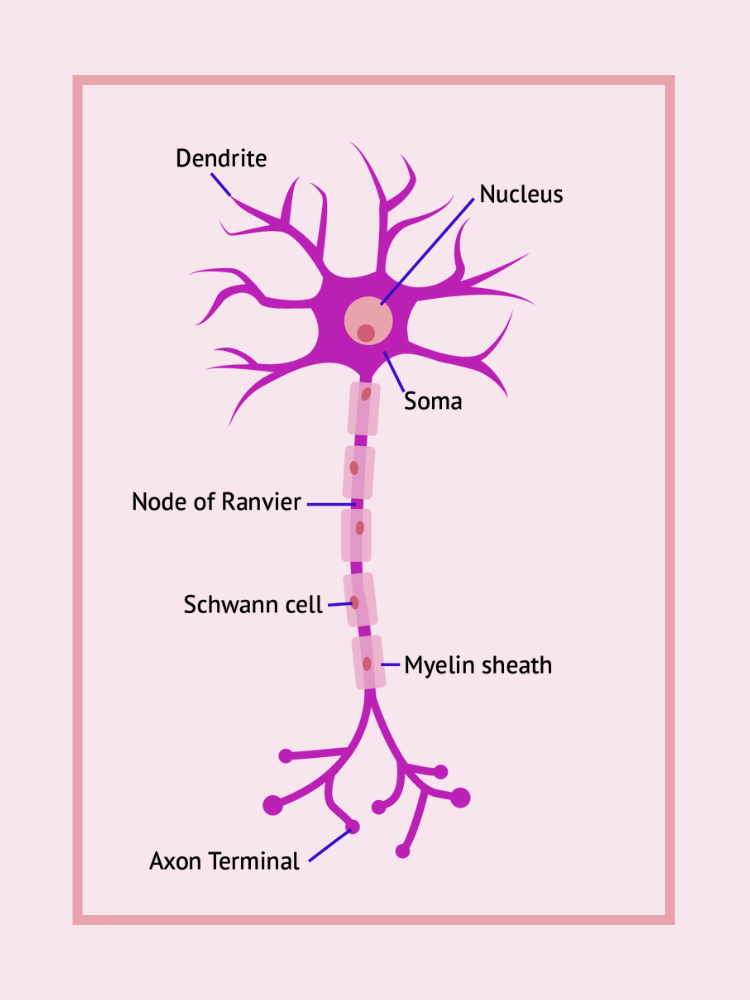
Illustration of a neuron
Illustration of a neuron
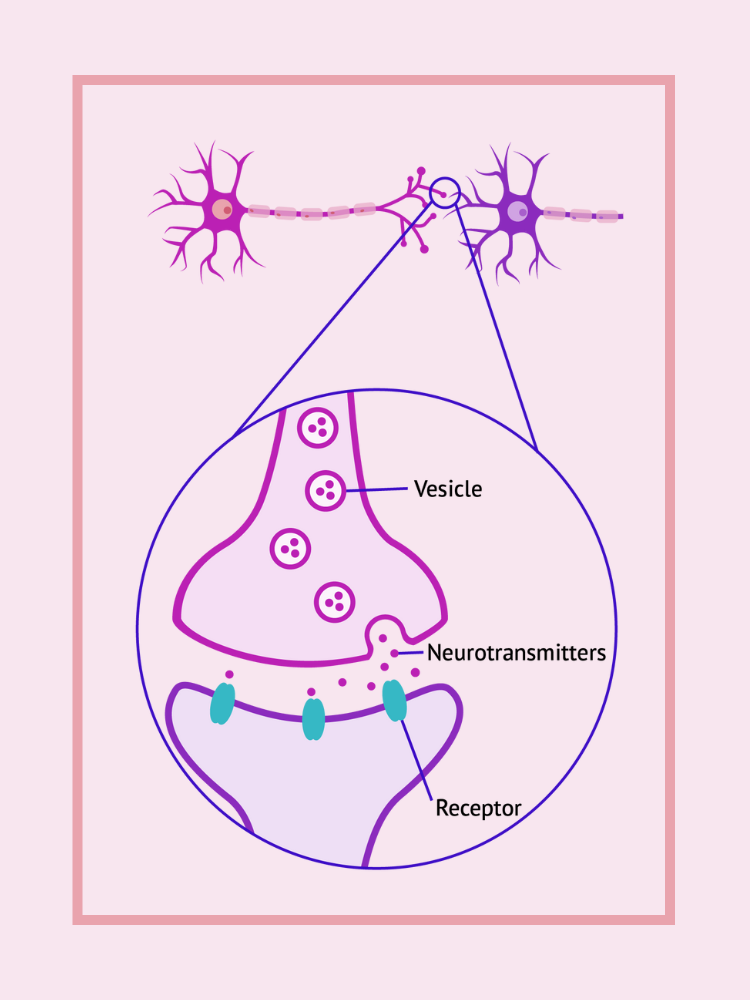
Illustration of a synapse
Synapse
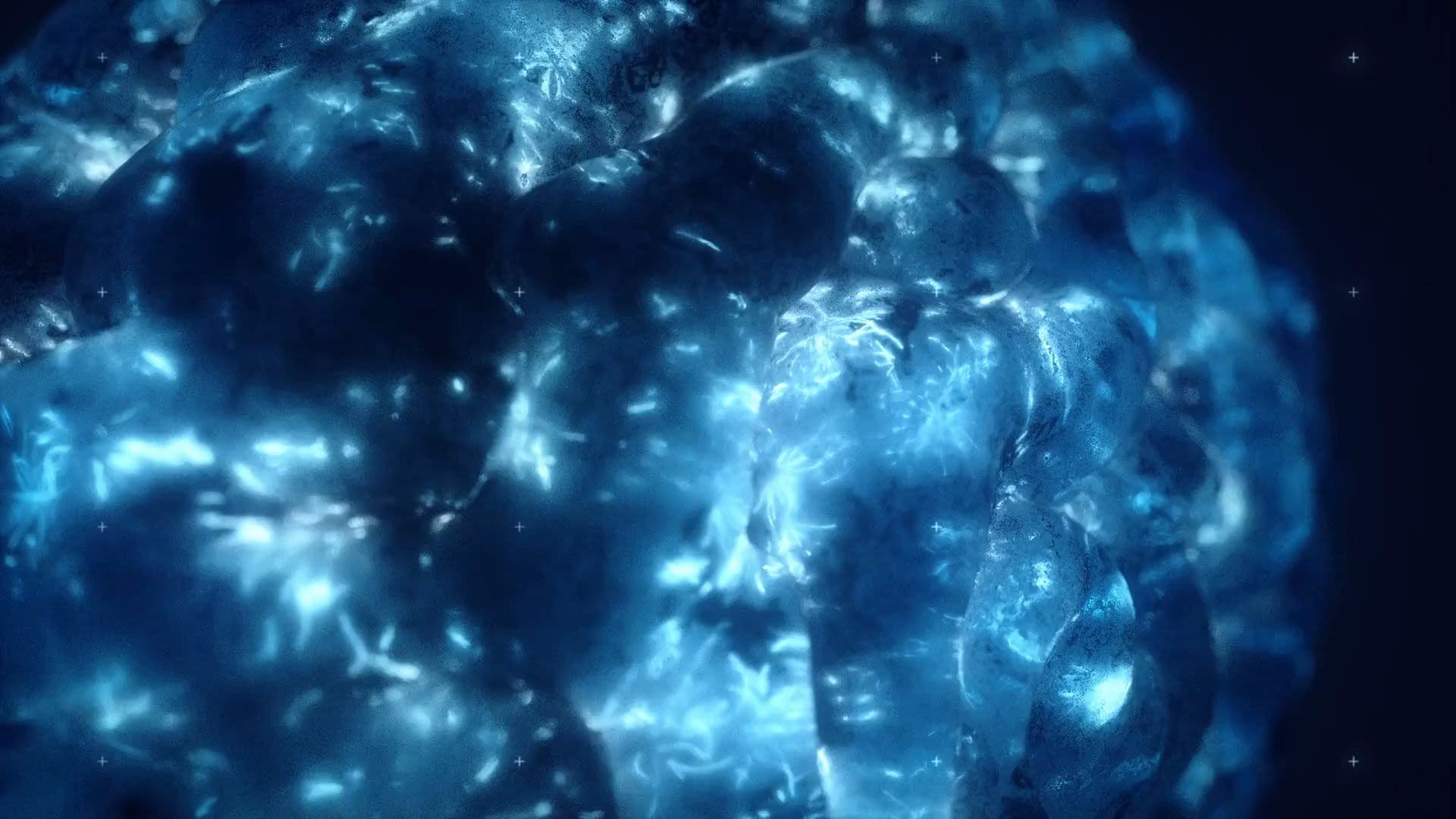
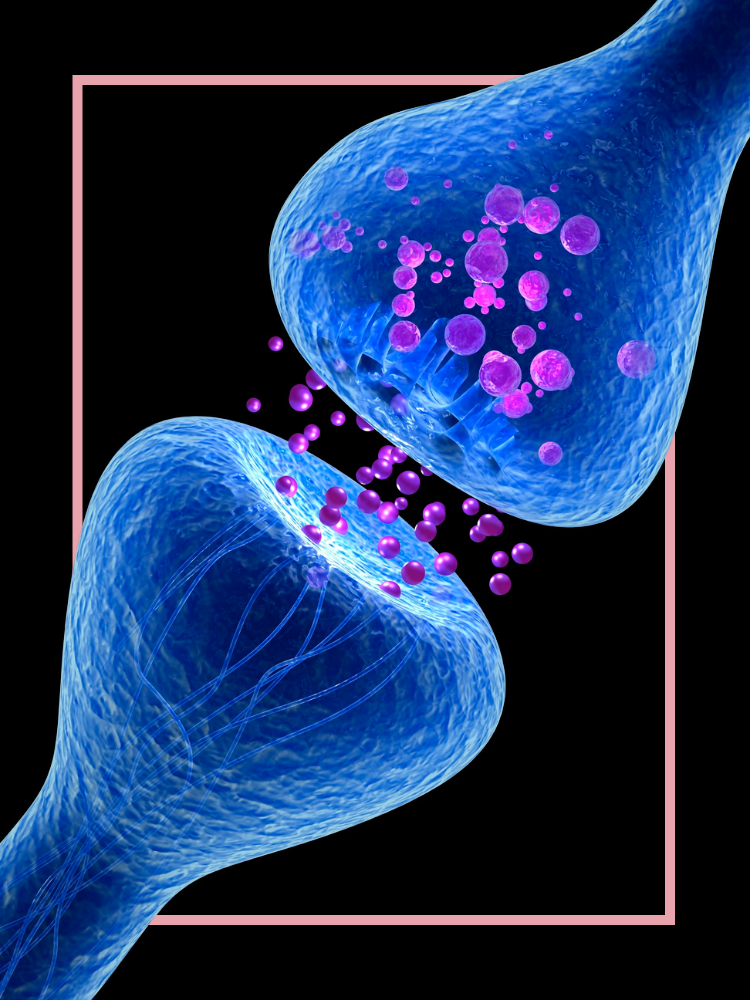
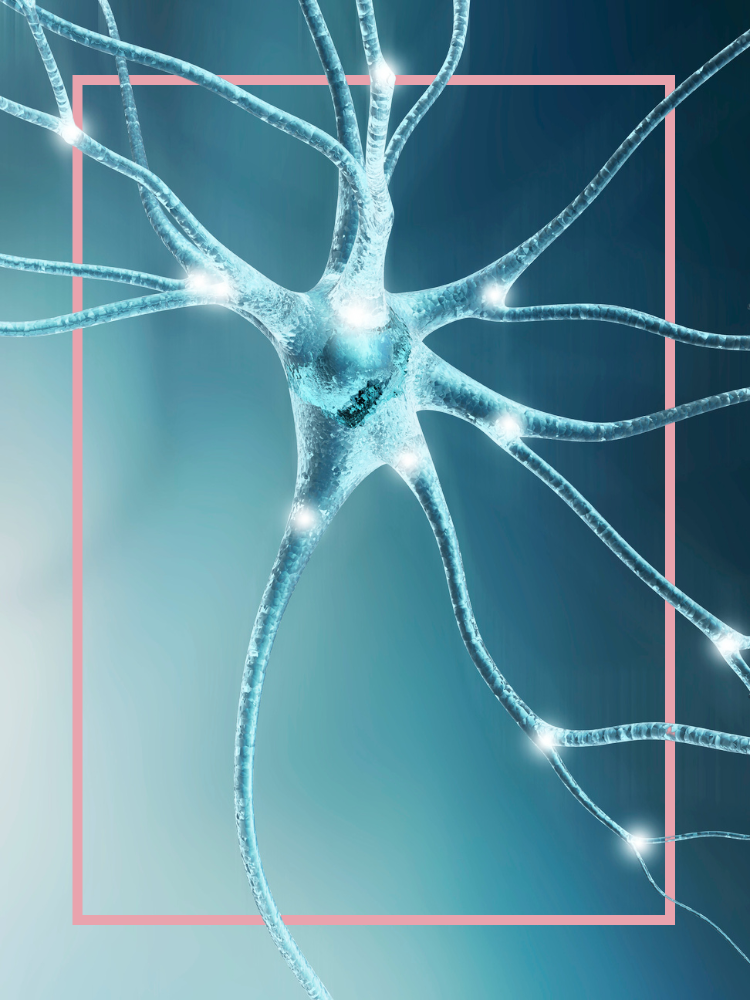
Neurotransmitters
Researchers from Experimental Psychology’s Social & Evolutionary Neuroscience Research Group explored the relationship between endorphins and enabling social cohesion, imperative for survival throughout our evolutionary history and vital for our health and well-being.
The team led by Dr Bronwyn Tarr looked at how musical behaviours such as singing and dancing release endorphins. They found that that moving energetically or moving in synchronisation can both make you feel closer to others, and lead to higher pain thresholds.
The study of neurotransmitters has led to substantial advances in our understanding of their impact on human health and behaviour, as well as neuropsychiatric disorders.
Neurotransmitters:
Neurotransmitters are chemical messengers which transmit signals across a chemical synapse or junction between two neurons. Examples of neurotransmitters in the brain include dopamine, serotonin and endorphins.
The neurotransmitter dopamine, long associated with reward-motivated behaviour in the brain, has been shown to play a key role in the human immune response. Researchers at the Kennedy Institute of Rheumatology at the University of Oxford demonstrated for the first time how human immune cells use dopamine, in specialised vesicles localised to the immunological synapse, to accelerate the immune response.
The collaborative research involving groups from Australia, Italy, Germany and the UK predicts that the neurotransmitter dopamine is used to accelerate antibody production in humans, which helps us to fight off infections more quickly. This work can now be used as a basis for the development of new therapies to treat immune diseases and improve vaccines.
‘This study has highlighted important parallels of cellular communication in the immune and nervous systems. Using state of the art facilities at the Kennedy Institute of Rheumatology we were able to visualise the location of dopamine containing vesicles and the consequence of dopamine communication across the immunological synapse.’
The dopamine system is the brain’s central hub for learning, and may play a role in shaping and optimising our decision-making process.
Dopamine neurons are active when we receive a reward, and when we anticipate that we are about to receive a reward, but until recently little has been understood about the mechanism behind this. Research from Professor Stephanie Cragg and her team, in collaboration with Associate Professor Ed Mann, from the Department of Physiology, Anatomy and Genetics, uncovered previously unknown observations about this mechanics behind this network.
‘Dopamine neurons dynamically change their firing rate over a large range of frequencies to signal different events related to reward prediction, anticipation, prediction errors and perhaps movement, so it is important that we understand how dopamine axons convert this activity into dopamine output. The axons of dopamine neurons are not passive cables that faithfully convert neuronal activity into dopamine release, but rather they contain active mechanisms that will significantly impact on the kinds of signals that dopamine neurons ultimately convey in the form of dopamine release.’
The group’s discoveries have the potential to further research into the role dopamine plays in diseases such as drug addictions and Parkinson's.
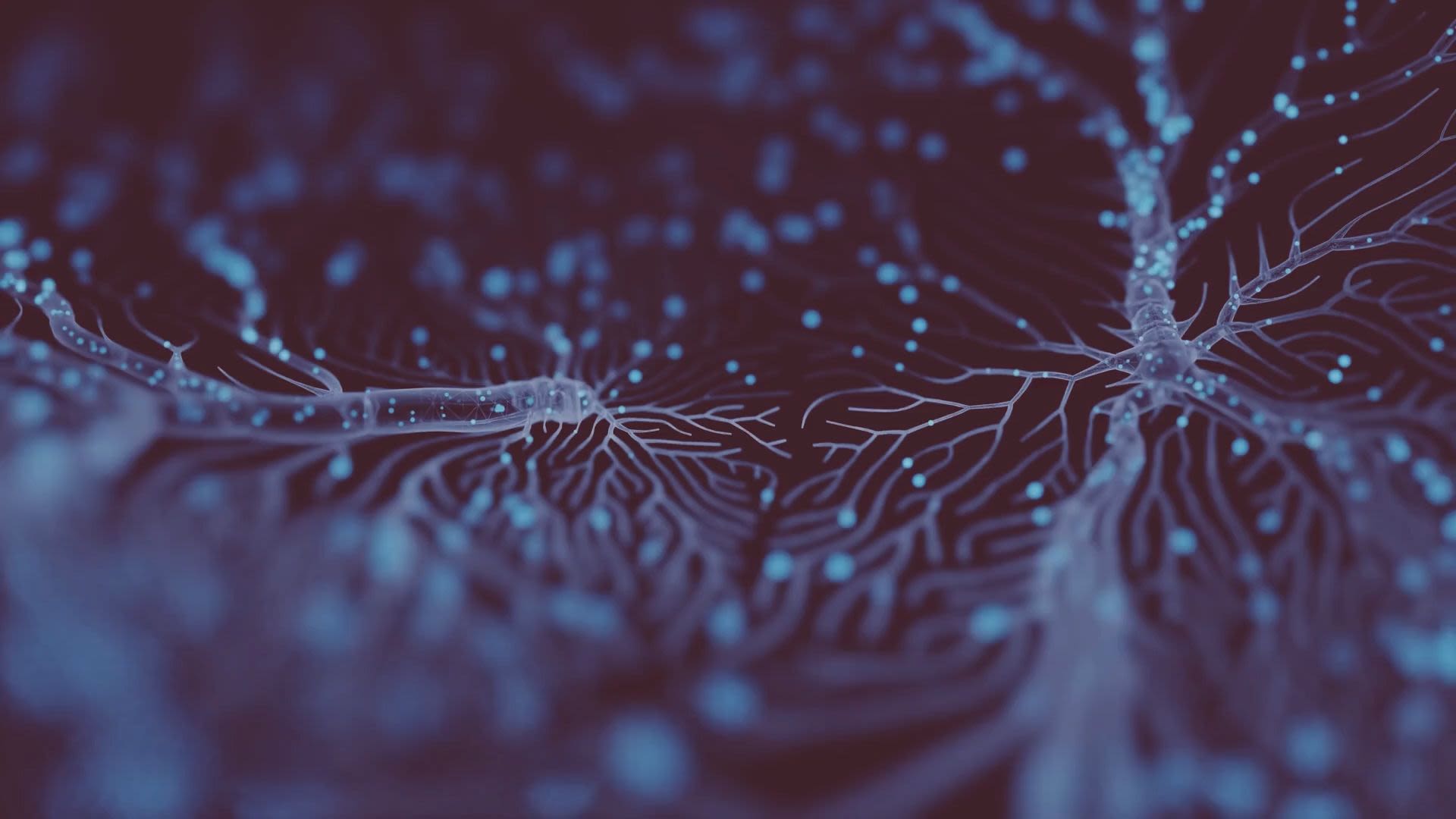
Consciousness and the brain
Understanding how the human brain functions, let alone how it generates thought and consciousness, is a monumental task.
Oxford experts are working to scan, model and understand the human brain and uncover the countless potential benefits for computing, society and healthcare.
The Computational Neurology Lab at the University of Oxford is taking on this challenge and has partnered up with hospitals, clinicians, psychiatrists, surgeons and several large data providers to build and test a wearable device that both captures and interprets multi-modal data.
The team maps neural activity and may hold the key to extracting sentiment, intent and even language, directly from neurological signals.
Researchers at the Nuffield Department of Clinical Neurosciences have received a prestigious Wellcome Innovator Grant for investigating the role of the pedunculopontine nucleus (PPN) – a brainstem nucleus – in human consciousness.
A collaboration between Neurosurgery, Engineering and Neurology, the study will involve a 10-subject first-in-man clinical trial of PPN stimulation in patients with minimally conscious state following brain injury such as trauma or stroke, to further develop the Deep Brain Stimulation investigational system called the ‘Picostim-DyNeuMo’
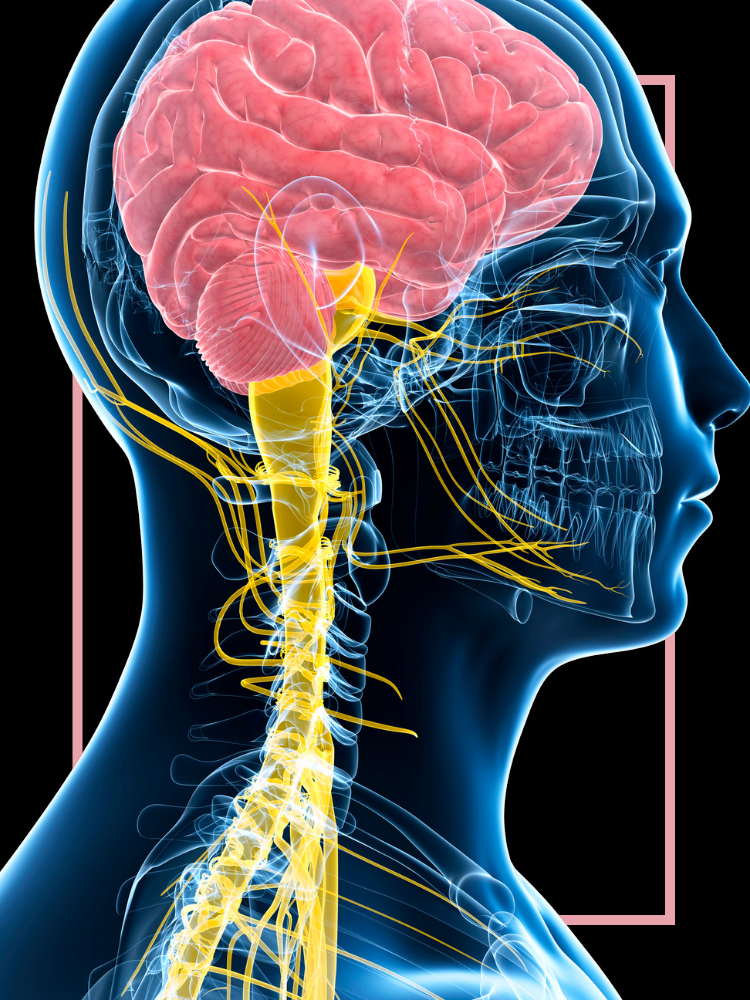
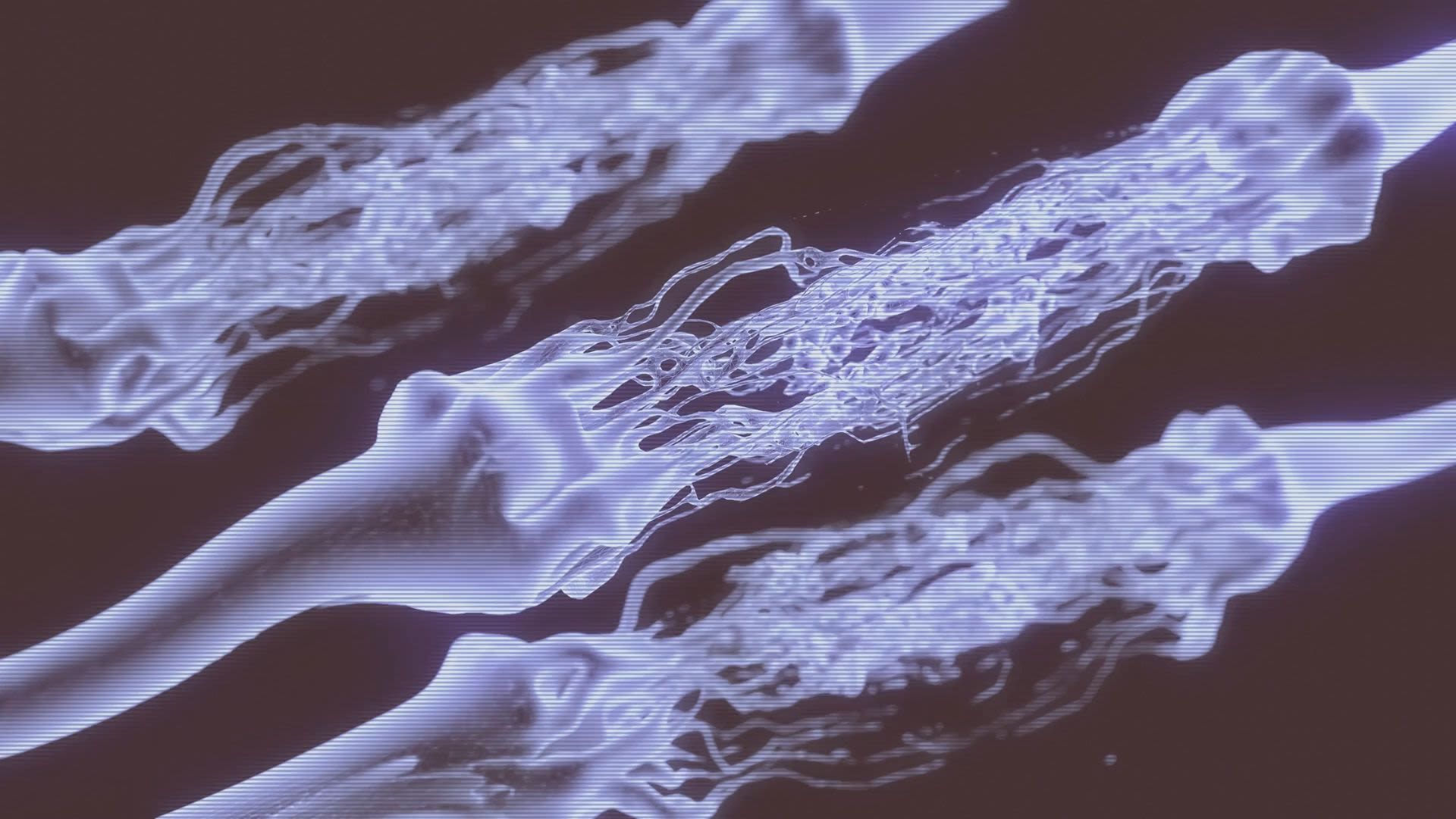
Future of the brain
Deep brain stimulation
Over 200,000 people worldwide to date have received DBS systems.
Deep brain simulation (DBS):
Deep brain stimulation (DBS) is an approved, safe, and effective treatment for patients with neurological conditions, such Parkinson's disease, with motor symptoms that cannot be adequately controlled with medication.
The MINDS feasibility trial is a collaboration between Associate Professor Alex Green at the Nuffield Department of Surgical Sciences, Professor Tim Denison at the Department of Engineering Science, and a UK-based bioelectronics technology company, Bioinduction Ltd, and involves a five-subject first-in-man clinical trial with the novel closed-loop brain pacemaker to target the pedunculopontine nucleus (PPN) stimulation in patients with multiple system atrophy (MSA).
The trial is being supported by the University of Oxford and the Oxford University Hospitals NHS Foundation Trust. Funding was provided from the UK government’s BEIS department through the Royal Academy of Engineering, the MRC Brain Networks Dynamic Unit, and the John Fell Fund at Oxford.
‘This is an exciting time for medical device research in the UK. In the past, often clinicians would explore new indications for existing technology whilst engineers would build new systems in parallel. This collaboration aims to integrate the development of innovative technology with exploration of mechanisms underlying disease states from an early stage and will therefore increase our understanding of disease at the same time as trying to treat it.’
Until recently the underlying mechanisms of DBS remained elusive.
A study led by a large international team, including Professor Morten Kringelbach from the Department of Psychiatry along with colleagues from Denmark and Spain, mapped the underlying whole-brain mechanisms for the use of DBS to alleviate symptoms of Parkinson's Disease.
The results of the study showed that DBS causes a global rebalancing of brain dynamics following high frequency stimulation in the subthalamic nucleus in patients with Parkinson’s Disease.
‘In general, the perspective of the present study is that we are now able to use whole-brain computational modelling to simulate the effects of brain interventions and predict the outcome. Longer term, we are hoping to be able to use these methods to personalize interventions for the benefit of individual patients.’
Brain implants
A number of start-ups are developing implantable systems that are able to listen to, interpret and speak with individual neurons within the brain. Their research is guided by recent advancements in our understanding of the human neural network, in combination with advancements in computing capability, mathematical modelling and machine learning.
Professor Newton Howard, Professor of Computational Neurosciences and Neurosurgery at the Nuffield Department of Surgical Sciences, discovered a functional optical layer in the human neocortex. This new insight into how the brain works may lead to uncovering causes of brain disorders, such as Alzheimer's disease, Parkinson's disease and epilepsy.
‘Understanding brain function and dysfunction requires a multidisciplinary approach and various methodologies ranging from biology to mathematics. One of the biggest challenges is decoding how the brain and its neurons are structured and how they function. This information is crucial for better understanding normal brain function and possibly uncovering causes of brain disorders.’
A spinout of Oxford University, ni2o, is now working on developing the necessary nanotechnology and optoelectronics to probe and drive this newly discovered optical layer, with the aim of treating neurodegenerative brain diseases and disorders.
Ni2o was founded by a team of entrepreneurs, technologists, and neuroscientists, including Professor Howard. Their Kinetic Intelligent Wireless Implant (KIWI) is a small, self-contained, wirelessly connected, wirelessly charged, AI-driven replacement for current deep brain stimulation (DBS) solutions. The device is implanted in a minimally invasive surgical procedure and records brain activity to determine therapeutic responses.
Researchers led by Professor Howard at the Oxford Computational Neuroscience Lab in the Nuffield Department of Surgical Sciences took a significant step towards using Artificial Intelligence (AI) to build an artificial brain.
The team successfully prototyped a nanoscale, AI-powered, artificial brain in the form factor of a high-bandwidth neural implant, with the potential to provide relief for millions of patients suffering from neurological, psychiatric and psychological disorders as well as degenerative diseases.
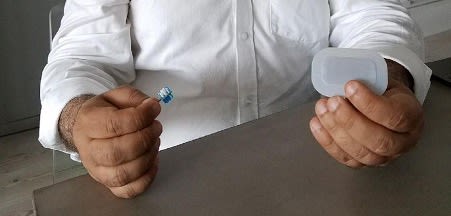
Professor Newton Howard holding parts of the implant device
Professor Newton Howard holding parts of the implant device
Scientists at the Oxford Martin School are taking a radical approach to treating brain injury, printing 3D brain implants generated from human cells to mitigate the effects of brain damage through injury or disease.
Leading this endeavour are Professor Zoltán Molnár and Professor Francis Szele of the Department of Physiology, Anatomy and Genetics, in collaboration with Professor Hagan Bayley of the Department of Chemistry, and Oxford Martin Fellow Dr Linna Zhou.
Through printing a variety of neurons and supporting cells, the team will develop layered ‘pre-organised’ printed neural tissue in vitro that will mimic the basic structure of cerebral cortex columns. They will then transplant the printed layered cortical tissue into animal models of traumatic brain injury, a condition which affects 5.3 million people globally.
‘How to produce the basic functional components of the brain, and integrate them meaningfully, is one of the biggest questions in cortical development and in neuroscience.’
Optogenetics
Optogenetics combines genetic targeting of specific cells or proteins with optical imaging or control of the targets, a technique that allows neuroscientists to read or write patterns of neuronal activity in the living brain.
Optogenetic control is a form of wireless communication in which the receiver of a wireless signal is fabricated from materials encoded in DNA.
Each nerve cell that switches on a specified gene produces an actuator protein, meaning the activity of the cell can then be controlled, simply by turning on an external light source.
Professor Gero Miesenböck, from the Department of Physiology, Anatomy & Genetics, was the first to demonstrate optogenetic control of neural activity and animal behaviour early in 2002, and subsequent work proved the utility of optogenetics for neurobiological research.
Professor Miesenböck is one of two scientists awarded the 2023 Japan Prize in the field of Life Sciences, rewarding significant contributions to society and major advances in scientific technology.
‘The Japan Prize is a major international prize that correctly recognises the outstanding discovery that Gero Miesenböck and Karl Deisseroth made in establishing a new methodology - optogenetics - using light sensitive protein to unravel neural circuits during complex physiological tasks. This has been one of the biggest advances made in neuroscience this century’
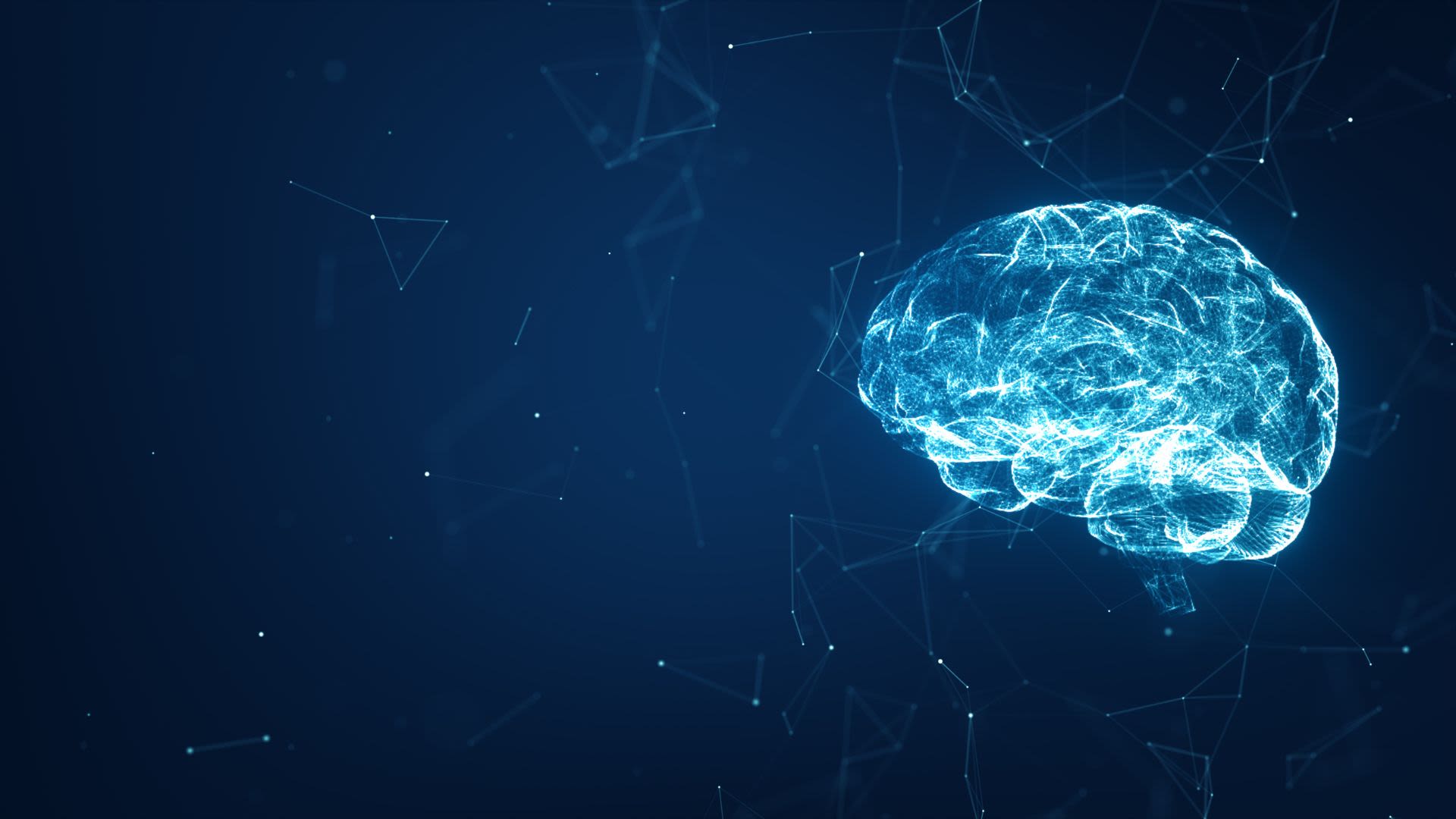
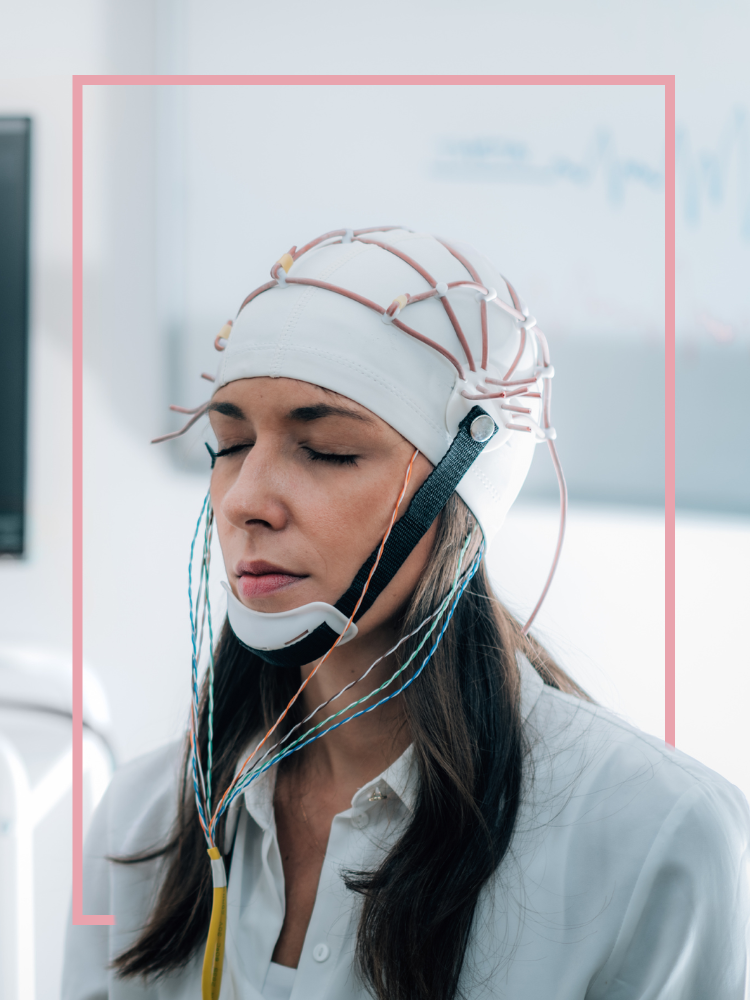
Ethical considerations
The risks and ethics of using something as invasive as deep brain stimulation, and other implanted devices, are being considered by researchers in this field.
Oxford neuroscientists teamed up with leading ethicists Professor Julian Savulescu, Dr Nicholas Shea and Professor Neil Levy from the Faculty of Philsophy’s Oxford Uehiro Centre for Practical Ethics to consider the issues new brain-boosting technologies could raise.
The treatment they investigated, transcranial direct current stimulation (TDCS), has been quite successful in treating psychological disorders such as anxiety and depression, as well as a tool for motor rehabilitation in stroke patients.
It is suggested that TDCS could be also used to enhance healthy people’s mental capacities, by making it easier for neurons in these brain regions to fire, enhancing the making and strengthening of connections involved in learning and memory.
‘At this stage, we need more research to understand better the risks and benefits, in specific populations, in real life. Any regulation should prevent misuse and abuse, but facilitate good research. This kind of technology could be as important as the internet and computing. Those are external cognitive enhancements. This is basic fundamental cognitive enhancement.’
Researchers at the Oxford Uehiro Centre for Practical Ethics are examining the ethical and legal questions raised by various neural interface technologies, including deep brain stimulation, following it’s success alleviating motor symptoms associated with neurological conditions.
Dr Hannah Maslen contributed to POSTNOTE Number 614, a research briefing advising the UK take the lead in developing responsible regulation and new applications for neural interfaces, due to the ethical questions they raise about privacy, fair access, assessing risks versus benefits, and attributing responsibility for actions.
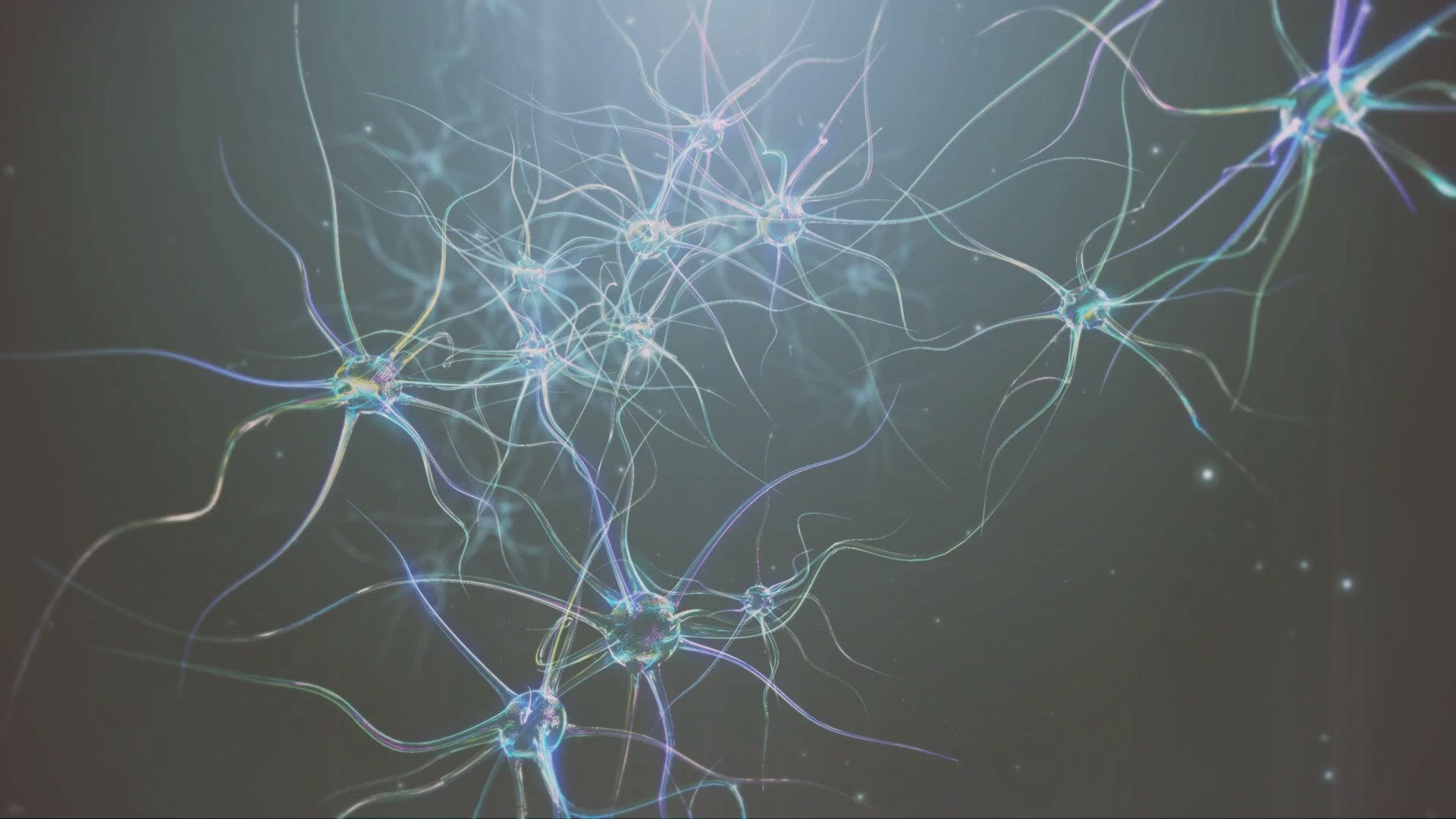